Island Mice Project
Overview
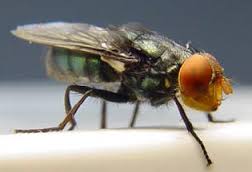
We can consider past eradications that did not use genetic engineering. Successful eradications using the sterile insect technique (SIT) have occurred with the screwworm (cochliomyia). Photo: Courtesy of Wikipedia
The use of genetic modification as a technique for eradicating mice from islands is currently being researched, and it could potentially be accessible in the future. To forecast issues that could arise from this, we should understand the ecological implications that this technology brings. Because this is something that does not yet exist, there are no empirical studies of what ecological effects might occur.
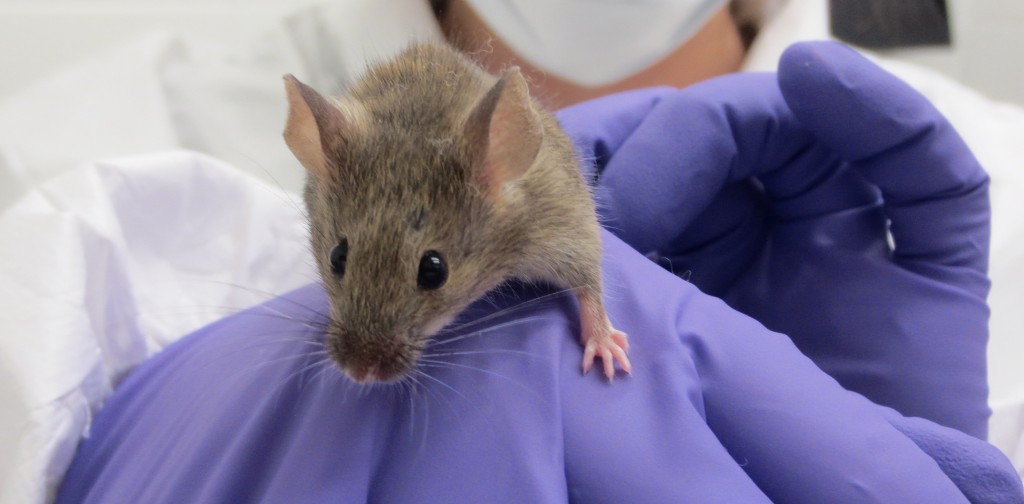
A mouse pup from a wild mouse population. What will occur when wild mice are housed together?
Photo: By Caroline Leitschuh
This ecological uncertainty creates justifiable concern. However, there are techniques that can help lower aspects of this uncertainty. In particular, we can make theoretical predictions based on established knowledge of population genetics, microevolution, and community ecology, and we can learn from experience in the genetic pest management of insects.
How is this Strain Created?
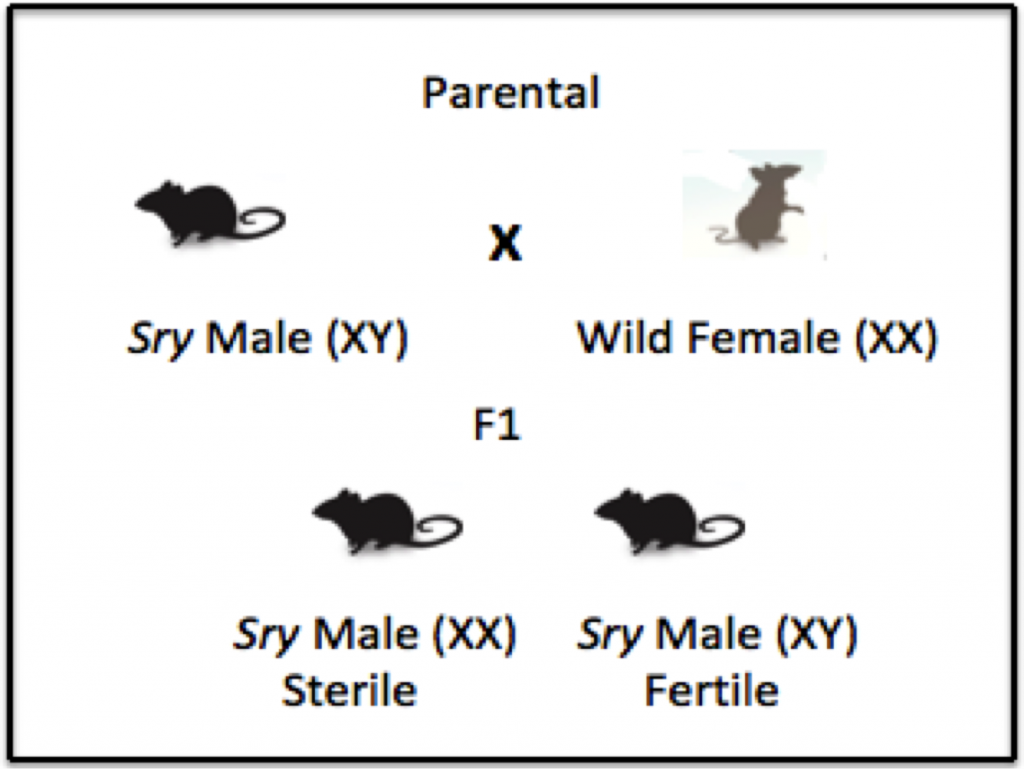
Depiction of how a population can become all male. Without males the population will decrease and possibly collapse. Figure By: Megan Serr
The method of genetic engineering that we are exploring as an option involves a specific rearrangement of the mouse chromosome. Foreign DNA would be used as a way to “cut” a gene of interest from one chromosome and “paste” it into another chromosome. The method we are exploring depends on how sex is determined in mice. Like humans, the sex of a mouse is determined by two chromosomes, the X and the Y chromosomes. Two X chromosomes lead to the development of a female mouse, and an X and a Y chromosome lead to the development of a male mouse. A gene on the Y chromosome, the Sry gene, is what determines the development of male characteristics.1 The transgenic strain that we are examining would have this Sry region moved to an autosome, or a non-sex chromosome. The Sry region will also be located in a section of an autosome with high meiotic drive – this means that the region is more likely to be inherited by offspring. Because the Sry would no longer be on the Y chromosome, both XX and XY offspring will receive the Sry gene and develop into physically male mice, though the XX males would be sterile. The XY males would be able to reproduce, continuing to pass on the translocated Sry region. In this way, a population of mice could be biased towards being predominantly male. As the population becomes predominantly male, less reproduction is possible, and over time the lack of reproduction will lead to a population decline. (See what could happen section)
References
- Genetics Home Reference. (2 June 2014). SRY. Retrieved from http://ghr.nlm.nih.gov/gene/SRY
How can this Gene Eradicate an Invasive Mouse Population?
Within the species (population level)
How would this ideally work?
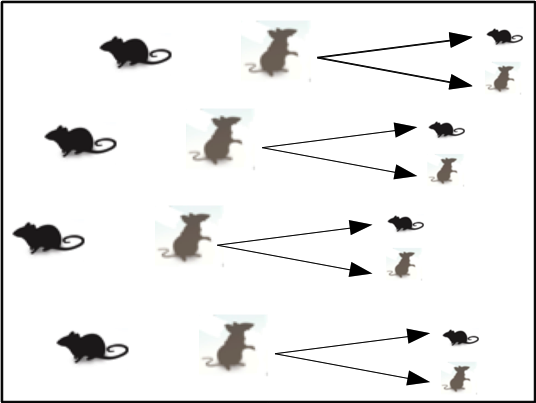
Population A has more mice in the second generation than Population B.
The main strategy of using genetic modification to reduce the population sizes of house mice is to change the sex ratio of offspring to produce relatively more males than females. With this occurring over multiple generations, a reduction in population size is predicted over time. Why would a simple distortion in the sex ratio lead to population decline? This can be answered by the fact that house mice are not naturally monogamous (unlike some other related mouse species).1 Thus, males have nearly an unlimited amount of reproductive potential, as long as fertile female mice are present. On the other hand, females must go through a gestation period after mating, limiting their ability to contribute their genetic information to future generations. Ultimately, this makes female mice the limiting factor in the change of population densities over time.
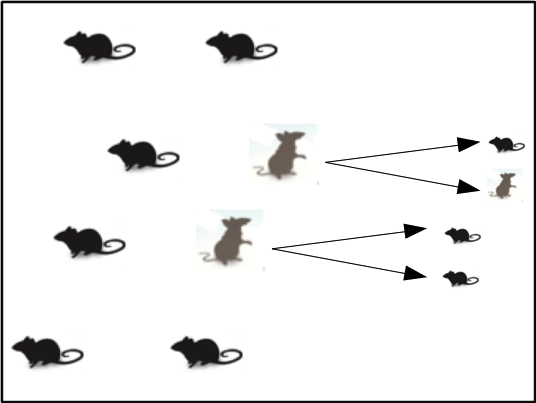
Population B only has fewer females than Population A. Therefore, the next generation will be smaller Population A with a 1:1 sex ratio.
To illustrate this point, consider the following comparison. We can imagine Population A consisting of 20 mice with 10 males and 10 females. Additionally, we can consider a second Population B consisting of 20 mice, but this time with 18 males and 2 females. For simplicity, we can imagine that females produce an average of 2 offspring that survive and reproduce in their lifetime. Thus, Population A will produce 20 new offspring while Population B will only produce 4 offspring. Despite both populations having identical population sizes, the difference in sex ratio ultimately leads to consequences in the next generation. Therefore, constructing a genetic complex that alters the natural sex ratio in favor of male offspring, would lead to a trend of decreasing the population size over time.
Why might this not actually happen?
As with other eradication strategies, the ultimate goal of releasing genetically modified mice should be to guarantee the complete removal of mice on the island. But there are two reasons why releasing genetically modified mice may not completely eradicate the island mice. As suggested by evolutionary theory, any organism in the wild faces two primary challenges in life: surviving and reproducing. These are referred to as natural selection and sexual selection, respectively, and they can both pose significant challenges for spreading a modified gene through a population.
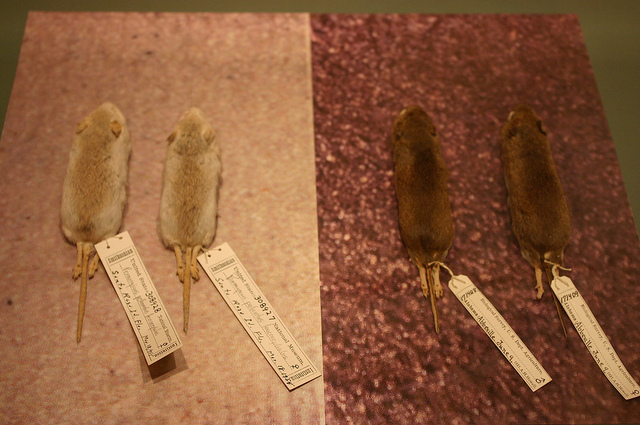
The two different coat colors here seen with the mice are from the process of natural selection.
Photo: By Ryan Somma
In terms of natural selection, an individual with a genetic modification is less likely to survive in the wild relative to one that is not.2, 3 When these genetically modified mice interact with unmodified mice, they must compete for resources and mating opportunities. Because of the relative decrease in fitness, genetically modified mice will not contribute as many of their genes to the next generation. Over time, this will result in the eventual loss of this genetic modification from the population.
In terms of sexual selection, wild mice might not be willing to mate with these unfamiliar genetically modified mice. This isn’t necessarily because the genetic modification itself makes the mouse unattractive; it can also occur because these new mice are just unfamiliar to native populations. Especially if mate choice is at all genetically or behaviorally linked, any non-island mice could easily be selected against over time.
Thus, ecological theory suggests it is unlikely that a single release of genetically modified mice will lead to eradication by itself. As the frequency of genetically modified mice decreases relative to non-modified mice, they would become less effective at population reduction over time. To combat this, a full eradication would likely require multiple releases.
What else could go wrong?
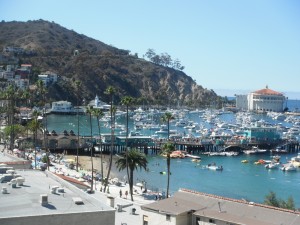
Many islands including Catalina island show here have heavy boat traffic.
Photo: By Elizabeth Pitts
A rather large concern from the ecology of genetic modification comes from the possibility of that genetic modification spreading beyond the intended population to other populations of the same species. Because the surrounding water is a boundary that prevents these mice from spreading, island ecosystems are ideal for this sort of technology. However, despite the isolation of islands, recall that the original invasive mice somehow got to the island in the first place (as most islands will have at least some boat or even aircraft traffic). Thus, the possibility of genetically modified escaping the island cannot be ignored. This might not be a large concern because, as explained above, the genetic modification would be unlikely to remain in the population after enough time.4 However, ecology has the tendency to lead to surprises. Strict inspection of everything that leaves the island during the eradication period can also be helpful in limiting the possible spread. Despite this, those considering this technology need to be well aware of the potential for these mice to spread beyond the island. If this technology were effective enough to eradicate an island with a single release (which it is not as it is currently proposed, but might be someday), it would have the alarming possibility to cause a rapid population decline on other islands or the mainland.
Beyond the species (ecosystem level)
Would a genetic modification spread to other species?
Unlike other methods of eradication (link to toxicants, mechanical traps, biological control, and other methods) genetic modification has the benefit of being species specific. Genes can usually only flow between organisms of the same species into the next generation of offspring. It is very unlikely for genetic material to be transferred between animal species unless we consider it over thousands of years.3 Thus, we can be fairly confident that the genetic modification will stay within the species of interest. However, especially if there are other related species within the ecosystem (such as other rodent species), it is a good idea to be aware of the unlikely, but existent, possibility of genes flowing between species.
Even if the possibility of genes flowing between species is very small, it might be significant concern for some. Because of the scientific complexities involved, more technical discussion of inter-species gene transmission can be found below. However, it is important to understand that while this scientific information might help quantify the amount of risk involved, it does not determine how much risk that people might find acceptable. Thus, this can easily become an issue of values instead of science.4 Both are necessary to determine if even a very small risk is worth it.
How else might the eradication affect other species?
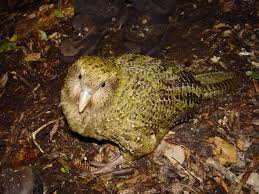
The Kokapo of New Zealand is critically endangered. Releasing more rodents to control wild rodents could increase the threat to other small and endangered animal populations.
Photo: Courtesy of Wikipedia
More likely, indirect effects on the rest of the ecosystem are possible when manipulating mouse population sizes. This technique would require an initial release of genetically modified mice onto the island. Thus, there will be a temporary but rapid increase in the population size relative to its previous state. In use of similar genetic pest management techniques for mosquitoes, this often requires a rather large increase in the population size.5 If the large number of mice on the island were already having a strong negative effect on the island, it is likely that a further increase in population size, even if only temporarily, could cause even more ecological harm. By devastating the plants and animals it consumes, outcompeting the animals that share these resources, and providing food for their predators, an increase in mouse population size might lead to negative consequences, including even a permanent extinction of other species, within the ecosystem.
This impact can be somewhat reduced if the genetically modified mice are released when the local mouse population is at a low point in its population cycle. In the end, even if this technique had fewer direct impacts on other species, it would still require an extensive assessment of how the mice indirectly interact with rest of the ecosystem. As with other eradication strategies, a whole-ecosystem approach should be considered.
References
- Brown, R. Z. (1953). Social behavior, reproduction, and population changes in the house mouse (Mus musculus L.). Ecological Monographs, 23, 217-240.
- Marrelli, M. T., Moreira, C. K., Kelly, D., Alphey, L., & Jacobs-Lorena, M. (2006). Mosquito transgenesis: what is the fitness cost? Trends in Parasitology, 22, 197-202.
- Snow, A. A., Andow, A., Gepts, P., Hallerman, E. M., Power, A., Tiedje, J. M., & Wolfenbarger, L. L. (2005). Genetically engineered organisms and the environment
- Pielke, R. (2007). The Honest Broker, Making sense of science in policy and politics. Cambridge University Press, U.K.
- Thredgill et al. Personal Communications and Genetic Pest management Course, North Carolina State University, 2013.
- Zavaleta, E. S., Hobbs, R. J., & Mooney, H. A. (2001). Viewing invasive species removal in a whole-ecosystem context. Trends in Ecology and Evolution, 16, 454-459.
Patents: What does it mean to Own Life?
So far in the United States, the fate of transgenic organisms has been determined primarily by judges and government officials, with very little input from the public at large. On this page, we review key court rulings that relate to genetically modified organisms. Elsewhere on the site, we discuss how these rulings affected public deliberations about genetically modified organisms, how these organisms are currently governed, and how they could be governed in the future.
Genetic engineering has spurred vibrant debates about what it means for an organism to be natural vs. unnatural, what differences there are between living things and technologies, what kinds of value different forms of organisms can have, and what obligations humans owe to the animals we use for various purposes. These questions continue to play out in the US patent system through debates about ownership and intellectual property.
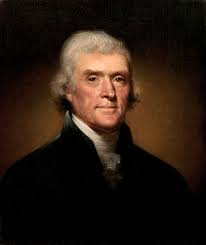
Thomas Jefferson is largely attributed to the formation of the patent process in the United States.
Photo: Courtesy of Wikipedia
United States patent law originated in a 1793 statute written by Thomas Jefferson, which states that patents may be awarded for “any new and useful art, machine, manufacture, or composition or matter, or any new or useful improvement thereof”.1 Aside from a single change that Congress made in the 1950s (replacing the term “art” with the term “process”), Jefferson’s original definition still stands.1 Awarding US patents for genetically modified organisms therefore depends on whether that organism, or the process that created it, is considered new enough, and different enough, from what has come before it–and on whether a person or organization believes that the organism will generate enough profits to justify the cost of the patent.
As historian Daniel Kevles 1,2 notes, Jefferson and his contemporaries would have been unlikely to envision that living organisms would be patented. In their view, plants and animals were neither machines nor manufactures, nor could these organisms be improved to create new forms of matter. The first attempts to treat living organisms as what we would now call intellectual property began towards the end of the nineteenth century, when nursery owners and horticulturalists sought to lay claim to profits generated by trees and plants that they claimed to have “originated” via selective breeding. These efforts were blocked by a landmark 1889 ruling by the US patent commissioner, who deemed it “unreasonable and impossible” to patent “the trees of the forest and the plants of the earth” (Ex parte Latimer, 1889 Dec. Comm’r. Pat. 123, 126, 46 O.G. 1638, 1639). This ruling contributed to the “product-of-nature” doctrine, which prohibits the patenting of products originating in the natural world.
Legal scholar Christopher Beauchamp argues that another approach was also developing in the late 19th and early 20th centuries: the test of “useful difference,” which deemed that “an isolated natural substance could be the subject of a valid patent. . . if the act of isolation rendered it greatly more useful than the product in its natural state”.3 Just over 100 years ago, arguments in the 1911 court case Parke-Davis & Co. v. H.K. Mulford Co. (189 F. 95 S.D.N.Y. 1911) spurred debates about the appropriateness and legitimacy of patenting medical compounds such as aspirin, adrenaline, and insulin–debates that foreshadowed contemporary controversies over the patenting of living organisms. Beauchamp suggests that the increasing prevalence of this “useful difference” approach may stem in part from the fact that the “product-of-nature” doctrine lacks a clear legal grounding. As he puts it, “Anyone looking for a historical ‘right’ answer on the product-of-nature question will be disappointed. For all the spirited attempts to impose consistency on the case law of natural subject matter, it remains a kaleidoscope of doctrine”.3
Patenting genetically modified organisms
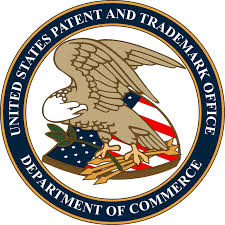
Seal of the United States Patent and Trademark Office
Photo: Courtesy of Wikipedia
Both the product-of-nature doctrine and the useful difference test converged in the landmark 1980 US Supreme Court case Diamond v. Chakrabarty. Ananda Chakrabarty, a scientist, sought to patent a live animal–a form of bacteria that he had genetically engineered to break down crude oil. Before Chakrabarty’s case, the US had issued only one patent in its entire history for a living organism: the purified yeast that Louis Pasteur had patented in 1873.1
The Patent Office denied Chakrabarty’s request for a patent based on the product-of-nature doctrine, arguing that because the bacteria were alive, they could not be patented. After multiple appeals, the US Supreme Court ruled against the Patent Office and in favor of Chakrabarty, whose attorney claimed that the bacteria were “much more akin to inanimate chemical compositions such as reactants, reagents and catalysts than. . .to horses and honeybees or raspberries and roses”–in other words, that questions about the patenting of living things were less relevant to bacteria than to higher life forms.1 However, just eight years later, their ruling gave precedent to the patenting of two much higher life forms: a genetically altered oyster as well as a transgenic mouse that had been modified to become more likely to develop cancer.4
Public deliberation?
Science and Technology Studies scholar Sheila Jasanoff 5 notes that another option was available to the justices who decided Diamond v. Chakrabarty. The court could have decided that Thomas Jefferson’s 1793 patenting language was an inadequate guide to distinguishing between living and nonliving matter. Had the justices taken this course of action, it would have been up to Congress to craft legislation defining when, where, how and why various forms of life could or could not be patented. This would have opened the door for American citizens to play a more active role in guiding these decisions, as their European counterparts did when faced with similar questions.5
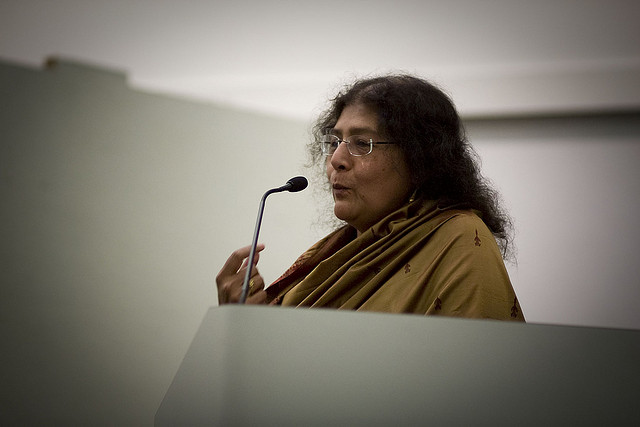
Sheila Jasanoff a Scholar in Science and Technology Studies (STS). Photo: Courtesy of Wikimedia.
As an example, Jasanoff cites the Supreme Court of Canada’s 2002 refusal to patent the transgenic mouse mentioned above, which had been modified to facilitate cancer research. Despite the fact that this mouse or similar ones had already been patented in Europe, the United States, Japan and New Zealand, Canada’s highest court argued that the legislative arena, not the judicial one, was a more appropriate forum to consider broad questions related to the ownership of biotechnological life forms.5
In the US, shifting the debate from the courts to Congress would have enabled a greater degree of citizen input because legislative branches are more accountable to the citizenry as a whole. Because members of Congress are elected rather than appointed, they have more incentive to take the opinions of voters into account. Scholars of democratic politics argue that there are substantive, normative, and instrumental reasons for involving citizens in decisions about contentious issues such as biotechnology.6,7 The substantive rationale is that citizens have valuable insights that will improve the quality of public policies.8 The normative argument notes that public consultation is fundamental to democratic government.8 The instrumental approach suggests that involving more people in the governance process makes the resulting laws and rules more legitimate.8
In the context of biotechnologies, the lack of meaningful opportunities to contribute to regulatory decision-making has undermined citizen confidence in risk assessments and in the governance system itself.9 The 1986 Coordinated Framework for the Regulation of Biotechnology Products, which now serves as the primary US oversight mechanism for genetically engineered organisms, was developed with little public consultation. Instead, White House officials relied primarily on technical advice from scientists to determine that the risks posed by genetically engineered organisms are no different from those posed by other kinds of organisms and products. In part because they wanted to avoid the controversies associated with genetically engineered organisms9, these officials chose not to advocate for new laws to govern these organisms. Instead, they created a framework based on existing laws including the Toxic Substances Control Act; the federal Insecticide, Fungicide, and Rodenticide Act; the Federal Food Drug and Cosmetic Act; and the Federal Plant Pest Act [Where do Genetically Modified mice fit Legally?].
References
- Kevles, D. J. (1994). Ananda Chakrabarty Wins a Patent: Biotechnology, law, and Society, 1972-1980. Historical Studies in the Physical and Biological Sciences, 25(1), 111–135. doi:10.2307/27757736
- Kevles, D. J. (2008). Protections, Privileges, and Patents: Intellectual Property in American Horticulture, the Late Nineteenth Century to 1930. Proceedings of the American Philosophical Society, 152(2), 207–213.
- Beauchamp, C. (2013). Patenting Nature: A Problem of History. Stanford Technology Law Review, 257, 257–311.
- Hurlbut, W. B. (2005). Patenting humans: Clones, chimeras, and biological artifacts. Science and Engineering Ethics, 11(1), 21–29. doi:10.1007/s11948-005-0052-x
- Jasanoff, S. (2005). Designs on nature: science and democracy in Europe and the United States. Princeton, N.J.: Princeton University Press.
- Cornwall, A. (2008). Unpacking “Participation”: models, meanings and practices. Community Development Journal, 43(3), 269–283. doi:10.1093/cdj/bsn010
- Gastil, J., & Levine, P. (2005). The deliberative democracy handbook: strategies for effective civic engagement in the twenty-first century. San Francisco: Jossey-Bass.
- Fiorino, D. J. (1990). Citizen Participation and Environmental Risk: A Survey of Institutional Mechanisms. Science, Technology, & Human Values, 15(2), 226–243.
- Kuzma, J., Najmaie, P., & Larson, J. (2009). Evaluating Oversight Systems for Emerging Technologies: A Case Study of Genetically Engineered Organisms. The Journal of Law, Medicine & Ethics, 37(4), 546–586. doi:10.1111/j.1748-720X.2009.00431.x
Where do Genetically Modified Mice fit Legally?
It is difficult to know for sure how a mouse that was genetically modified for eradication purposes would be regulated. In many ways, such a mouse stretches the limits of current US policies related to biotechnologies. Here, we review those policies and suggest potential courses of action that could be taken in overseeing the potential release of this mouse and other genetically modified mammals.
As we mention on this page, the current US system for overseeing the potential release of genetically modified organisms is called the Coordinated Framework for the Regulation of Biotechnology Products. It was created in 1986 by officials at the White House Office of Science and Technology Policy. They directed federal agencies to oversee the potential release of these organisms by relying on existing laws: the Toxic Substances Control Act; the Federal Insecticide, Fungicide, and Rodenticide Act; the Federal Food Drug and Cosmetic Act; and the Federal Plant Pest Act.1
The coordinated framework is built on the assumption that the risks posed by genetically engineered crops were essentially the same as the risks posed by crops that had not been engineered.1 In keeping with this view, the framework does not consider the processes through which genetically engineered organisms are created. Instead, it focuses on the final product: the organism itself.
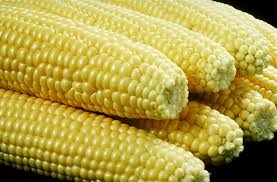
Corn is a common genetically modified crop in the United States
Photo: Courtesy of Wikipedia
Focusing on final products rather than processes can raise ethical concerns in relation to the manipulation of mammals and other higher life forms.1 These issues are particularly salient in the cloning process. To clone cats or cattle, scientists can harvest immature eggs from a single female animal’s ovaries and transplant them into a laboratory petri dish, where the eggs grow and mature.2 However, for reasons that are not fully understood, this process is much more difficult in working with dogs. For instance, to create Snuppy, the world’s first cloned dog, researchers in South Korea implanted 1,095 cloned embryos into 123 female dogs. Only two of these embryos were born, and only one of the two survived.2 In a 2005 editorial that commented on this process, the editors of the highly respected science journal Nature wrote that “It is unlikely that even the most obsessive pet owner would contemplate preparing more than 100 failed pregnancies for just one successful birth…In such circumstances, the cloning of dogs…remains ethically indefensible”.3
Genetically modifying mice for the purpose of eradicating invasive populations would not cause similarly high numbers of lost or damaged embryos. A more likely possibility is that mice that have been modified according to the Sry method might develop mutations such as Swyer syndrome, a condition in which animals with a pattern of chromosomes normally found in males develop female bodily characteristics.
United States laws do offer protection to animals used in cloning, genetic modification, and other kinds of research. In total, more than 50 federal laws have been passed to safeguard these animals, the most significant of which is the 1966 federal Animal Welfare Act. In 2002, Congress amended the Act to specifically exclude mice and rats from its protections, despite the fact that they are used in more experiments than any other kind of mammal.2 However, laboratory rodents continue to be protected through the 1985 Health Research Extension Act, which established the Institutional Animal Care and Use Committee (IACUC), a self-regulating body established by federal law that oversees and evaluates every aspect of animal care in institutions that use laboratory animals for research purposes. For example, in addition to establishing standards for the care and housing of laboratory mice, rats, and other animals, IACUC mandates that scientists must provide for the well-being of these animals by administering painkillers or anesthesia as appropriate.
If and when they are taken out of the laboratory and released into the wild, the protections afforded to genetically modified organisms could change substantially, depending on what type of animal it is and how it is used. For example, different legal rules apply to pets, livestock, and laboratory animals.3 Similarly, wildlife are offered different protections depending on where they are located, whether they are considered endangered species, whether their primary habitat is terrestrial or aquatic, and whether they are considered a risk to human health or safety.3
Similarly, the Coordinated Framework for Biotechnology directs different federal agencies to review genetically engineered organisms depending on what category the organism falls under. Because the Framework is comprised of laws that existed prior to the development of genetic engineering, these categories are sometimes an awkward fit. For example:
- The US Department of Agriculture (USDA) regulates organisms categorized as “plant pests”–in other words, those that could potentially become an invasive pest like a weed.4
- The US Environmental Protection Agency regulates organisms categorized as “pesticides.” This category refers to:
- “proteins or other chemicals that serve as a natural defense against pests,” or
- “bacteria, fungi, viruses, protozoa, or algae, whose DNA has been modified to express pesticidal properties” 5
- The US Food and Drug Administration (FDA) regulates organisms categorized as “new animal drugs,” which the agency defines as “an article (other than food) intended to affect the structure or any function of the body of … animals”.6
We argue that mice that have been modified for the purposes of eradication could potentially fit into any or all of these categories. For example, although unlikely, it is possible that this type of genetically modified mouse could spread beyond the area in which they are introduced, or that the modified genes they carry could be transferred to other species. In this case, the mice or their transgenes could act as a kind of invasive pest, disrupting ecosystems in unintended ways. It is also possible, though unlikely, that Sry mice would be considered a kind of pesticide, given that they are being created with the goal of curbing populations of invasive pests. A more likely possibility is that the Sry mice could be seen as a kind of animal drug, given that they are being created with the goal of affecting the structure and function of invasive mouse populations.
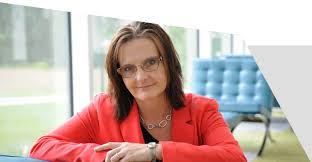
Jennifer Kuzma Center Co-Director of the Center on Genetic Engineering and Society at NCSU. Photo: GES Center at NCSU
A key problem associated with regulating genetically engineered mice through any of these categories is that attaching any one label would severely limit the scope of risk assessments and public deliberations. For example, the International Risk Governance Council calls for regulatory agencies to consider issues ranging from biosafety and biosecurity to intellectual property and ethics when regulating genetically engineered organisms.7 Calling the Sry mouse a “pest” or a “pesticide” or a “drug” seems an inadequate way of accounting for these many different kinds of risk because each of these categories oversimplifies scientific complexities.8 As policy scholars Jennifer Kuzma and Todd Tanji note, “many policy issues related to oversight … are [not] appropriately framed by ‘risk.’ For example, ethical issues are embedded in environmental risk assessment… but are not themselves typically considered risk issues. The choice of intellectual property regime (open source, security classification, patent protection) can affect biosecurity and economic development, as well as pose difficult ethical dilemmas about ‘owning life.’” 7
It’s possible that even a regulatory framework that looked beyond such narrow definitions of risk would render important questions invisible. For example, what would it mean to make genomics a science of extermination, and to create a mammal that functions as its own extermination device? What, if any, obligations would humans owe to such a creature? Where, when, and why would modifications be considered acceptable or unacceptable? When, if at all, would it be acceptable to replace a naturally occurring population with a genetically modified one?
References
- Kuzma, J., Najmaie, P., & Larson, J. (2009). Evaluating Oversight Systems for Emerging Technologies: A Case Study of Genetically Engineered Organisms. The Journal of Law, Medicine & Ethics, 37(4), 546–586. doi:10.1111/j.1748-720X.2009.00431.x
- Anthes, E. (2013). Double Trouble. In Frankenstein’s cat: cuddling up to biotech’s brave new beasts (First edition., pp. 56–78). New York: Scientific American / Farrar, Straus and Giroux.
- A dog’s life. (2005). Nature, 436(7051), 604–604. doi:10.1038/436604a
- USDA APHIS. (2014, March 19). Am I Regulated? Retrieved from http://www.aphis.usda.gov/wps/portal/aphis/ourfocus/biotechnology?1dmy&urile=wcm%3apath%3a/aphis_content_library/sa_our_focus/sa_biotechnology/sa_regulations/ct_am_i_reg
- US EPA, O. of P. P. (2012, January). EPA’s Regulation of Biotechnology for Use in Pest Management | Pesticides | US EPA. Retrieved from http://www2.epa.gov/regulation-biotechnology-under-tsca-and-fifra/epas-regulation-biotechnology-use-pest-management
- US FDA. (2013, April 23). Genetically Engineered Animals – General Q&A. WebContent. Retrieved from http://www.fda.gov/AnimalVeterinary/DevelopmentApprovalProcess/GeneticEngineering/GeneticallyEngineeredAnimals/ucm113605.htm
- Kuzma, J., & Tanji, T. (2009). Unpackaging Synthetic Biology: Identification of Policy Problems and Options (SSRN Scholarly Paper No. ID 1451425). Rochester, NY: Social Science Research Network. Retrieved from http://papers.ssrn.com/abstract=1451425
- Stirling, A. (2010). Keep it complex. Nature, 468(7327), 1029–1031. doi:10.1038/4681029a
How Much Would it Cost?
The cost of developing the strain is difficult to calculate, and there are many unknowns. As the development of the mouse is still in its first stages and it is expected to take years to create a strain that could potentially be used for a conservation context, many costs have not yet been settled. Some possible sources of monetary costs are development of the mouse, behavioral and genetic testing of different prototypes, maintaining a colony, facilities and supplies costs, and personnel costs. Many of these costs vary case by case and cannot be anticipated this far in advance.
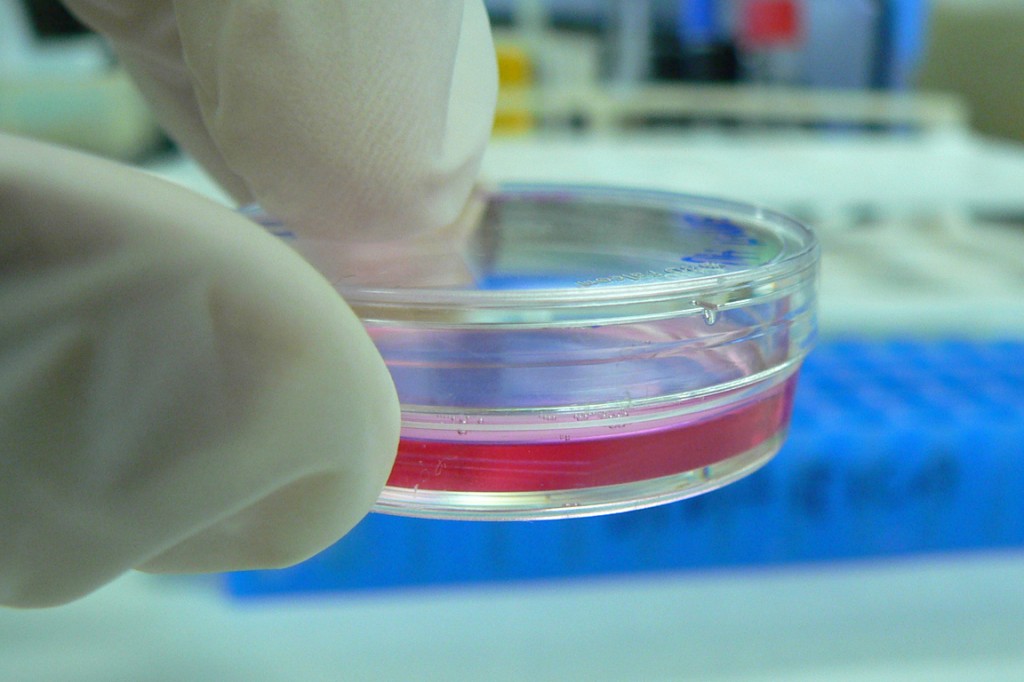
Cell cultures are commonly used to examine genetic alterations to tissue. Photo: Courtesy of Wikipedia
We can predict some of the specific costs associated with developing a transgenic lab strain, which is the first step in developing the Sry mouse strain. Some general procedures necessary for creating transgenic mice, such as injections of genetic material to alter the genome, typically cost $2-4000 per injection, and it takes many trials to get the correct genomic alterations.1 Other procedures will be needed in the process, but cannot be anticipated here as they may vary by institution and by lab, and we do not have access to that information at this time.
Another cost is colony maintenance fees. For example, at NCSU the cost per cage of mice can range from $0.20 to $1.50 per day, with basic medical and testing procedures being $20 per animal per procedure. Additional maintenance services, such as weaning or euthanasia, are $5 each time.2 These costs are specific to NCSU, and may vary by institution or by the level of care needed for a strain.
An additional cost to consider would be patenting the strain. While there would be monetary costs associated with a patent, there are also complications related to how a genetically modified organism would be classified under patent laws. For more details, see the section on patents.
Costs for implementation as population control
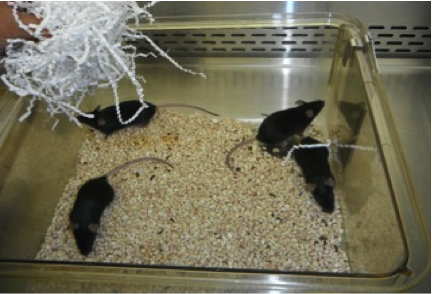
Stain C57BL/6 commonly known as Black Six Mice housed at NCSU.
Photo By: Megan Serr
Compared to the use of toxicants the deployment of the Sry mouse would be simpler. It would only take a few people to release the mice onto an island, and they would need to be on the island for a shorter period of time. However, there would still be costs. One cost would be in purchasing the required number of mice. Black 6 Mice, the standard lab mouse for genetic manipulation, are sold to researchers for $18-66 depending on the age and sex.3 The Sry mouse is being developed in a Black 6 background, meaning that the Black 6 is the “base” for the genetic manipulations. However, the final cost for the Sry mouse will be dependent on a host of other factors, including ease of colony maintenance and patents, and so cannot be predicted at this time.
References
- Oregon Health and Science University. (2013). Services & Costs.
- Biological Resources Facility. (2013). Forms. Retrieved from http://brf.sciences.ncsu.edu/forms-2/
- The Jackson Laboratory. (undated). C57BL/6J. Retrieved from http://jaxmice.jax.org/strain/000664.html